Connecticut’s trees and forests provide a multitude of social, environmental, and economic benefits every day. The Extension Forestry Program of the University of Connecticut Cooperative Extension System provides education for natural resource professionals, elected and appointed officials, volunteers and private woodland owners who care for this valued resource and landscape. Extension educators—in cooperation with many organizational partners—seek to improve the health, care, diversity, and management of Connecticut’s trees and forests. Educational efforts reflect the wide diversity of the state’s forested landscape and ownership—from small-scale private woodlands, land trusts and woodland cooperatives to state forests; from street trees, town greens and parks to municipal watersheds.
The UConn Extension Forestry program works statewide to address forest stewardship issues. We support woodland owners, communities and Connecticut Forest Practitioners with information and educational programming. UConn Extension Forestry partners with public agencies such as CT-DEEP Forestry Division and non-profit organizations such as the Connecticut Forest and Park Association.
Extension Forestry Programs include educational offerings for woodland owners such as the Coverts Project and the Master Woodland Manager program, activities designed to benefit communities such as the Stormwise Initiative, and professional educational efforts for CT-Certified Forest Practitioners. Extension Forestry contributes the CT Urban Forestry Council, the CT Forest Stewardship Committee, the Oak Resiliency Project and the Northeast Forests and Climate Adaptation Network.
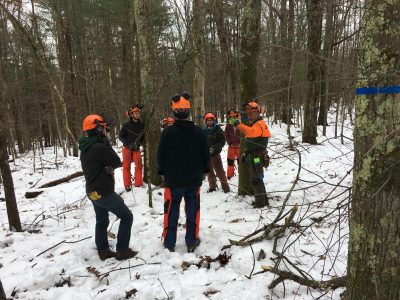
The Slow Storm: Tree and Stand Mortality in CT
The Slow Storm: Tree and Stand Mortality in CT during 2018 from drought, insects and diseases.
by Thomas E Worthley, UConn Associate Extension Professor, Forestry
During the last decade most tree-related front-page-newsworthy stories resulted from disruptions related to severe storm events that caused power outages, transportation disruptions and property damage that folks will long remember. Such events are sudden and dramatic, are certainly newsworthy and result in visible changes in our landscapes and neighborhoods. Less sudden and dramatic, but perhaps more intensively and extensively altering our wooded landscapes visibly and ecologically, is the slow and relentless “perfect storm” of weather patterns, invasive insects and opportunistic pathogens the last few years causing severe and extensive tree mortality, most noticeably in eastern CT. We are witnessing a landscape-scale change in forest species composition, age structure and stand condition such as not been experienced in a generation.
During the early summer of 2018 it became apparent that numerous trees throughout eastern and central Connecticut did not produce leaves this spring, having died sometime during the winter. It’s not unusual to lose a tree or two to natural causes at any time of year, but the massive scale and extent of oak tree (Quercus spp.) mortality during the winter of 2017 to 2018 was striking and concerning. The combination of drought conditions during 2015 through 2017 followed by widespread and intense gypsy moth (Lymantria dispar) infestations and defoliations in 2016 and 2017 is the primary cause. Many trees already stressed by drought conditions upon being defoliated simply were not able to produce sufficient carbohydrate reserves to survive. In some places a secondary mortality phenomenon is also evident. Early and mid-season browning of leaves on individual trees that leafed-out and seemed fine during spring of 2018 indicates that other pests or diseases continue to affect previously stressed trees, the main pathogens responsible being two-lined chestnut borer (Agrilus bilineatus), a native pest, and armillaria fungus, also known as “shoestring” fungus (Armillaria mellea). Both of these agents are known to opportunistically attack stressed trees.
Combined with the anticipated loss of ash trees (Fraxinus spp.) in our area due to the invasive emerald ash borer (Agrilus planipennis) moving across Connecticut, the sheer numbers of LARGE standing dead trees throughout the state presents what might be described as a slow-moving environmental disturbance. For some property owners the word “disaster” might be more appropriate, given the value of standing timber losses or the expenses associated with removals of large dead trees near homes.
A long period of detection sampling and information dissemination raised awareness about emerald ash borer with professionals, elected officials and members of the public, so we’ve been somewhat prepared for it. But the sheer degree and extent of oak tree mortality in central and eastern CT was unexpected and unprecedented and has overwhelmed many homeowners, Tree Wardens, foresters and others.
Implications of a sudden shift in forest composition and structure
What can woodland owners in affected areas expect to happen next? At this time some continued mortality from two-lined chestnut borer and armillaria fungus is anticipated and gypsy moth egg masses remain abundant in some spots. A wholesale loss of oak forests from the landscape is not expected, but stand structure and composition, habitat features and wildlife populations, and future stand growth patterns will be different.
- Many mature oak stands will become young oak stands, in upland settings where the oak canopy was dominant and the losses severe. A strong acorn crop in 2016 combined with abundant advance oak regeneration (seedlings that have been present for many years) at many upland sites will ensure a future oak forest at those locations. Landowners can encourage an oak component by means of forest improvement practices that eliminate competition.
- Some stands exhibiting partial oak mortality will transition to mixed-species stands. Much of the southern New England forest resource is comprised of “even-aged stratified mixtures”, in forestry jargon, where forest canopies are arranged in layers. The uppermost part of the canopy is occupied by oaks and other species that are not-so-tolerant of shade, and just beneath these crowns reside the more-shade-tolerant maples, beech and birches. In many cases these individuals can be expected to grow into and occupy canopy gaps left by dying oaks. It will be up to landowners to decide whether that is a desirable future condition.
- Habitat conditions are changing and wildlife populations will compensate. Birds that require large areas of unbroken forest canopy and animals that depend on abundant acorns will move to places where those conditions still exist. Observers can expect to see more birds that utilize canopy gaps and early successional structure, along with rabbits, mice, other rodents and ground-nesters. Insect-eating birds will find abundant forage, predators will enjoy numerous perching sites and as dead trees decay and topple over during the next few years, amphibian and reptile populations might benefit from unique habitat and food sources associated with decaying wood.
- Invasive species will invade even more. Near roads, fields, power lines and other forest-fragmenting features, where invasive species such as Japanese barberry and oriental bittersweet are already an issue, even partial canopy loss will worsen the problem. Ticks will thrive in these places. Management activities that control invasives will yield long term benefits.
- Hikers and hunters will need to look up! Constantly. Anyone making recreational use of woodlands in affected areas MUST be aware that dead branches and limbs can and will drop at any time under any conditions. Temporarily closing some popular recreational sites to the public might be advisable.
- Think twice before acting on the temptation to salvage that dead tree. The risk of brittle broken limbs falling and causing injury to a person cutting a dead tree is extremely high. If a dead tree needs to be removed, and you are not properly equipped and trained, the advice of a professional is essential. (Besides, we’ve already mentioned the tree is an awesome perching site and insect buffet!)
“Forest”, in all its variations, is the natural vegetative cover for most of southern New England, indeed, it’s estimated that 75% of Connecticut is under some sort of tree canopy. The forest is also resilient, relentless, creative and patient. Regardless of what we humans do to the land, trees will, ultimately, find a way to grow back and as we observe the effects of this “Slow Storm” we can be encouraged to remember this. While we can’t control the effects of slow or sudden storms, we can observe and enjoy the dynamics of re-growth, watch the changes that take place, help to encourage what’s desirable and control what’s not, slow down and be part of the process.
Forests and Climate Change
Southern New England Forest Management in an Era of Climate Change
A Position prepared by the Yankee Division of the Society of American Foresters
Purpose: This document reports the best available scientific findings and management strategies related to forests and carbon sequestration and storage in southern New England. This information will help guide development of public policy for forest management in this region. Forests are central to our history, identity and way of life. The forests of southern New England provide critical ecosystem services including water quality, biodiversity, carbon sequestration and storage, recreation, and human health, important products, and productive employment, while providing solace and sense of place. Climate change, coupled with New England’s land use history and increasing human population, has heightened the need to responsibly manage these forests for multiple uses including sequestration and storage of atmospheric carbon.
Scope: This statement outlines the ways in which a spectrum of forest stewardship strategies in southern New England contribute to climate change mitigation and adaptation, provide services and products for society, and sustain resilient forests for future generations.
Position
It is the position of the Yankee Division of the Society of American Foresters (Yankee SAF) that active forest management, grounded in science, is essential to maintaining and promoting resiliency and ecosystem services. Such management will:
1) Promote carbon sequestration and storage (Evans and Perschel 2009, McGarvey et al. 2015);
2) Provide additional ecosystem services including air and water pollution mitigation (Cardinale et al. 2012);
3) Provide locally sourced and sustainable wood products that substitute for more carbon intensive materials (e.g., wood instead of concrete, biomass fuels instead of fossil fuels) (Rudell et al. 2007);
4) Reduce forest fragmentation, mismanagement, and conversion to non-forest both locally and globally; and
5) Improve biodiversity and the capacity of ecosystems in southern New England to withstand and adapt to the impacts of climate change.
Issue
The issue of forests and carbon is complex and increasingly important. Carbon uptake (i.e., sequestration) occurs in growing forests, generally peaking in early stand development, but sometimes continuing at high rates through late-successional stages, particularly in structurally complex forest systems (Bormann and Likens 1979, Keeton et al. 2007, Keeton et al. 2011). Carbon storage occurs in the biomass of forested systems and in long-lasting wood products, such as lumber.
Wood products store carbon and help to offset the need for extraction and production of non-renewable, carbon-intensive materials, such as concrete, steel and petroleum-based plastics. Locally and regionally produced wood products have a relatively smaller carbon footprint due to lower transportation costs and are sourced from well-regulated forests (Kittredge et al 2002, Ashton et al. 2012).
Forest management, including timber harvesting, is consistent with goals of promoting long-term carbon sequestration and storage. Management practices continue to adapt as we gain a better understanding of the relationships between forestry and atmospheric carbon. Reducing harvest frequency and favoring high levels of structural retention, for example, can sequester up to 57% more carbon (Nunery and Keeton 2010). Reforestation also increases carbon sequestration (Rhemtulla et al. 2009). In urban areas this would also improve quality of life through other ecosystem services (Nowak and Greenfield 2008). Managing for a variety of values and uses on a long-term timescale using peer-reviewed forest science and a holistic understanding of the forest systems ensures that southern New England forests continue to capture and store carbon, maintain ecosystem functions and services, and decrease global deforestation and fossil fuel use. While reserve-based management is appropriate in some places, sustainable timber harvesting in most forests best serves human needs in the long term. Meeting these objectives will require a full suite of conservation strategies working together, including both sustainable harvesting and reserve-based management (Foster et al. 2017).
Background
The Importance of Forests
Forests are central to our history, identity, and way of life. The health of our forests will strongly influence our collective future. Forests renew the air we breathe and filter the water we drink. We rely on forests for a host of renewable raw materials for products from maple syrup and medicinal plants to fuel and lumber. Forests provide habitat to wildlife, beneficial insects and plant species. Forests prevent erosion, build soil, store nutrients, and sequester and store carbon. Forests also provide the joy of birdsong, inspiration, and renewal of spirit.
Forest Disturbance and the Need for Resilience
Disturbances play an important role in structuring the forested landscape and are vital for functions including regeneration. Disturbances range in type, size, frequency and intensity (Turner et al. 1998, Lorimer and White 2003). Variation in post-disturbance abundance and spatial arrangement of live and dead trees impacts species composition and carbon storage dynamics (Franklin et al. 2002, Seymour et al. 2002, Birdsey 2019).
While the most common natural disturbances in Southern New England forests are wind and ice storms, impacts of invasive insects and fungal diseases are increasing. Fire as a disturbance has only recently declined and could become influential again. Fire has a long and varied history in New England with its frequency, intensity, severity, and extent heavily influenced by changes in land use practices (Patterson and Sassaman 1988, Brose et al. 2014, Lorimer and White 2003, Hurteau et al. 2011, Janowiak et al. 2014). Anthropogenic disturbance (e.g., harvesting and silvicultural action) has also been an influential driver of forest condition, and response to decades of forest management is one of the largest factors shaping current forest conditions (Duveneck et al. 2017).
Climate change in the Northeast is altering ecosystem disturbance regimes (Evans and Perschel 2009). Changes vary seasonally and include increases to average temperatures, heavy precipitation events and drought, and decreases in snowfall and snowpack (Janowiak et al. 2018). Forest composition and condition models show varying responses to changes in climate and natural disturbance regimes (Tang and Beckage, 2010, Rustad et al., 2012). The impacts may happen at such a rate that the recovery of the forest ecosystem cannot keep pace (Liang et al., 2017), or cause substantial loss of species richness and diversity (Iverson and Prasad, 2001). At the same time, the landscape is facing loss of forests through conversion to other land uses (Kittredge, 2009, Olofsson et al. 2016).
Resiliency – a forest’s capacity to recover function after a disturbance – is critically important for sustaining forest ecosystems in this era of rapidly changing climatic conditions. Resiliency enables the forest to maintain, restore or enhance ecosystem services, including carbon sequestration and storage, following disturbances. Informed forest management and protection of forestland from development provides the opportunity to maintain or improve resiliency by retaining connectivity, increasing complexity and maintaining or enhancing diversity across forested landscapes (Catanzaro and D’Amato, 2019).
Forest Carbon
Forested ecosystems provide a valuable ecosystem service by storing and sequestering carbon, reducing atmospheric CO2. Temperate forest ecosystems have been widely acknowledged as a carbon sink (Ashton et al. 2012); U.S. terrestrial forests offset ten to thirty percent of annual U.S. CO2 emissions (Houghton 2003).
Trees and forest vegetation sequester carbon from the atmosphere through the process of photosynthesis. Carbon is stored in various pools including live and dead aboveground biomass, belowground biomass, woody material and leaf litter, and soil (Fahey et al., 2005; Catanzaro and D’Amato, 2019). Amounts of sequestered and stored carbon are dynamic – constantly fluxing between and within pools as forests and land-use change over time. Decades of research illuminate the variety of factors driving forest carbon sequestration and storage dynamics. Stand age is strongly predictive of aboveground biomass in the northeastern U.S., with other variables, including ecoregion and conifer composition, accounting for 25-33% of variability (Keeton et al. 2011). Disturbance, both natural and anthropogenic, is also a driving factor of carbon sequestration and storage dynamics (Birdsey et al. 1997, Duveneck et al. 2017).
Reports of carbon sequestration and storage vary widely due to these factors. In the Northeast, biomass (i.e., stored carbon) generally increases over time (Barford et al. 2001, Hadley and Schedlbauer 2002, Keeton et al. 2011), but can exhibit decline in different stand conditions or due to stressors (Fahey et al. 2005).
The rate in forest carbon uptake (i.e., sequestration) in the Northeast is declining (Birdsey et al. 2019), as has been observed in maturing forests (Bormann and Likens 1979, Keeton et al. 2007). However, managing for complex forest structure, as often found in primary and mature or old-growth secondary forests, can yield an increase or maintenance in net carbon sequestration (Luyssaert et al. 2008, Nunery and Keeton 2010).
The carbon stored in wood products adds to the complexity of carbon accounting. Hardwood flooring, dimension lumber, and plywood are forms of stored carbon and should be accounted for as such. Furthermore, the use of these products avoids carbon emissions from the extraction and production of more carbon-intensive materials such as vinyl, carpet, concrete, and steel (Oliver et al. 2014). Wood utilization and technology continue to improve the production of wood products and increase associated carbon storage (Tollefson 2017). Cross-laminated timber (CLT) is capable of replacing concrete for multi-story buildings (Robertson et al. 2012). A life cycle assessment of the four-story John W. Olver building at the University of Massachusetts found that the use of CLT and other wood products instead of concrete and steel reduced the building’s global warming potential by 13% (Gu and Bergman 2018). Substituting wood for steel and concrete in new buildings world-wide would reduce global CO2 emissions by 14 to 31% (Oliver 2014) and interest in this technology is rising (Struck 2019).
Sequestration in the forest and carbon emission offsets associated with wood products from sustainable forest management are critical components of carbon management. Research continues to increase our understanding and must guide forest practitioners to improving the capacity of this vital resource.
Sustainable Forest Management and Timber Harvesting
Sustainable forest management is a “dynamic and evolving concept, which aims to maintain and enhance the economic, social and environmental values of all types of forests, for the benefit of present and future generations” (FAO 2020). Yankee SAF strongly supports the practice of sustainable forest management that includes both limited reserves and responsible harvesting as the best way to ensure that forests continue to provide a wide array of benefits. FAO lists the following climate change mitigation and adaptation actions for forests:
Carbon sequestration enhancement by silvicultural practices
Carbon stock conservation by preventing deforestation, implementing reduced impact logging, and pest control
Substitution of wood products for steel, concrete, aluminum, and plastic
Reducing the vulnerability and strengthening the adaptive capacity of trees and forests.
Sustainable forest management can accelerate development of complex structure in northeastern U.S. forests (Keeton 2006), making it possible for early successional canopies to support the complex functioning and biodiversity seen in late-successional or old-growth forests (Donato et al. 2012). Reducing harvesting frequency (Curtis 1997), increasing rotation lengths (Harmon and Marks 2002, Ryan et al. 2010), and encouraging post-harvest structural complexity (Keeton 2006, Franklin et al. 2007, Swanson 2009, Puettmann et al. 2009) have been found to increase stand-level carbon storage. Maintaining adequate stocking of large trees (Stephenson et al. 2014), while also allocating growing space for younger trees can promote higher rates of stand-level carbon storage and sequestration (D’Amato et al. 2011). These practices can also strengthen forest resiliency. Each parcel’s unique species composition, forest structure, and landscape position must be evaluated to determine its vulnerability to disturbance and its role in benefiting present and future generations.
Sustainable forest management promotes diversity of species, ages, sizes, and spacing of trees, improving overall forest resilience. A gypsy moth outbreak that kills large oak trees will not harm tulip trees. A hurricane or tornado may flatten mature trees and not damage saplings that bend more readily. A drought can weaken or kill overcrowded trees but has less impact on trees freed from competition through active management.
Managing forests to promote resiliency and greater carbon storage is stand-specific. Sustainable forest management considers many different tree and site characteristics to determine the most suitable actions to meet the goals of forest management. The effects of certain management prescriptions on carbon sequestration and storage, for example, are dependent on stand age characteristics. Reducing harvest frequency more effectively increases carbon sequestration in uneven-aged New England stands than in even-aged stands (Nunery and Keeton 2010). Retaining such biological legacies as large old trees also promotes diversity by sustaining many organisms and critical ecosystem functions, such as soil stabilization, nutrient retention and recycling, and resilience to disturbance (Franklin et al. 2007, Hanson et al. 2012). Generally, silvicultural treatments that maintain a large proportion of mature trees maintain or increase aboveground carbon storage (D’Amato et al. 2011).
Sustainable forest management that includes harvesting reduces the volume of dead wood that will release carbon due to decay (Hoover and Stout 2007). The carbon in durable wood products such as plywood, framing, flooring and furniture is stored much longer than the carbon in dead trees (Russell 2014). In southern New England, the volume of wood in trees that die naturally is over three times that contained in harvested trees (Oswalt et al. 2019).
Durable wood products are more carbon-efficient than alternative products, in addition to storing sequestered carbon that would otherwise be released back to the atmosphere through decay. In addition to the benefit from the carbon stored in durable wood products, there is less carbon released from harvesting and manufacturing wood products than from mining non-renewable resources and manufacturing products from them (Bergman et al. 2014). Many studies have documented that one of the key carbon sequestration benefits of active forest management is the substitution of products made from wood for those made from steel, aluminum, or concrete (Oliver 2014, Woodbury and Wightman, 2017).
Forest products are important to our local economy. In 2013, Southern New England's forest-based economy accounted for an estimated $5.8 billion dollars in gross regional output and provided employment to approximately 28,525 individuals (Northeast State Foresters Association 2015). In addition, revenue generated from the sale of forest products helps encourage keeping forests as forests and limiting their conversion to non-forest uses.
A resilient forested landscape is comprised of a variety of forest conditions. Sustainable harvesting and management to preserve old forests each result in the storage of significant amounts of carbon. Minimally disturbed forests provide critical habitat for some species and are invaluable for scientific research. Forest management that includes harvesting can proactively and intentionally create or enhance habitat for the myriad vertebrate and invertebrate species that depend on young forests or forests with heterogenic structure (DeGraaf and Rudis 1992, DeGraaf et al. 2005). Sustainable forest management that includes harvesting yields additional benefits for useful, renewable products, reduced carbon emissions, and important aspects of resilience that preservation does not.
Resilient, vigorous, functional and diverse forests are critical for continuing our way of life in southern New England. The disturbance regime that our forests experience has changed due to the loss of some species (including apex predators), the introduction of others (especially invasive species), and a changing climate. Sustainable forest management maintains and enhances ecosystem function and resiliency so that the forest resource continues to meet societal needs. Water quality, soil integrity, carbon capture, diverse wildlife habitat, forest products, recreational opportunities, and aesthetic beauty can be maintained or increased. We have the opportunity and the responsibility to be a part of the solution.
References
Ashton, M.S., Tyrrell, M.L., Spalding, D. and Gentry B. (eds.) (2012) Managing Forest Carbon in a Changing Climate, Springer Science+Business Media, pp 77-99.
Page 7 of 11
Barford C.C., Wofsy S.C., Goulden M.L., Munger J.W., Pyle E.H., Urbanski S.P., Hutyra L., Saleska S.R., Fitzjarrald D., and Moore K. (2001) Factors Controlling Long- and Short-Term Sequestration of Atmospheric CO2 in a Mid-latitude Forest. Science 294:1688–1691.
Berlik, M.M., Kittredge, D.B., and Foster D.R. (2002) The Illusion of Preservation. Harvard Forest Bulletin No. 26.
Bergman R., Puettmann M., Taylor A., and Skog K.E. (2014) The Carbon Impacts of Wood Products. Forest Products Journal 64:220-231.
Birdsey R., Mickler R., Sandberg D., Tinus R., Zerbe J and O'Brian K, eds. (1997) USDA Forest Service Global Change Research Program Highlights: 1991-1995. Gen. Tech. Rep. NE-237. Radnor, PA: U.S. Department of Agriculture, Forest Service, Northeastern Forest Experiment Station. 122 p. https://doi.org/10.2737/NE-GTR-237.
Birdsey R.A., Dugan A.J., Healey S.P., Dante-Wood K., Zhang F., Mo G., Chen J.M., Hernandez A.J.; Raymond C.L., and McCarter J. (2019) Assessment of the influence of disturbance, management activities, and environmental factors on carbon stocks of U.S. national forests. Gen. Tech. Rep. RMRS-GTR-402. Fort Collins, CO: U.S. Department of Agriculture, Forest Service, Rocky Mountain Research Station. 116 pages plus appendices.
Bormann, F.H. and G.E. Likens, G.E., (1979) Pattern and Process in a Forested Ecosystem. Springer Verlag, New York. pp. 253.
Brose, P.H., Dey D.C., and Waldrop T. (2014) The Fire - Oak Literature of Eastern North America: Synthesis and Guidelines. NRS-GTR-135. Newtown Square, PA: U.S. Department of Agriculture, Forest Service, Northern Research Station. https://doi.org/10.2737/NRS-GTR-135.
Cardinale et al. (2012) Biodiversity loss and its impact on humanity. Nature 486:56-67.
Catanzaro P., and D’Amato A. (2019) Forest Carbon, University of Massachusetts Amherst.
Catanzaro P., D’Amato A., and Huff E.S. (2016) Increasing Forest Resiliency for an Uncertain Future. University of Massachusetts Amherst.
Curtis, R., (1997) The role of extended rotations. In: Kohm, K., Franklin, J. (Eds.), Creating a Forestry for the Twenty-first century: The Science of Ecosystem Management. Island Press, Washington, DC, pp. 165–170.
D’Amato, A. W., Bradford, J. B., Fraver, S., and Palik, B. J. (2011) Forest management for mitigation and adaptation to climate change: insights from long-term silviculture experiments. Forest Ecology and Management, 262(5), 803-816.
DeGraaf, R.M. and Rudis D.D. (1992) New England wildlife: habitat, natural history, and distribution. USDA Forest Service Gen. Tech. Report NE-108. 491 p.
DeGraaf, R.M., Yamasaki M., Leak W.B., and Lester A.M. (2005) Landowner’s guide to wildlife habitat. University of Vermont Press. Burlington, VT. 111p.
Donato, D.C., Campbell, J.L., Franklin, J.F. (2012) Multiple successional pathways and precocity in forest development: can some forests be born complex? Journal of Vegetation Science 23(3): 576-584.
Page 8 of 11
Evans and Perschel (2009) A review of forestry mitigation and adaptation strategies in the Northeast U.S. Climatic Change 96(1):167-183.
Fahey T.J., Siccama T.G., Driscoll C.T., Likens G.E., Campbell J., Johnson C.E., Battles J.J., Aber J.D., Cole J.J., Fisk M.C., Groffman P.M., Hamburg S.P., Holmes R.T., Schwarz P.A. and Yanai R.D. (2005) The Biogeochemistry of Carbon at Hubbard PM Brook. Biogeochemistry 75, 109–176. https://doi.org/10.1007/s10533-004-6321-y
FAO (2018) Climate Change for Forest Policy-Makers. Food and Agriculture Organization of the United Nations. FAO Forestry Paper 181 v2.0. http://www.fao.org/3/CA2309EN/ca2309en.pdf
FAO (2020) Sustainable Forest Management, Food and Agriculture Organization of the United Nations, http://www.fao.org/forestry/sfm/en/
Foster, D.R. (1992) Land-use history (1730-1990) and vegetation dynamics in central New England, USA. Journal of ecology: 753-771.
Foster, D., et al. (2017) Wildlands and Woodlands, Farmlands and Communities, Broadening the Vision for New England. Harvard Forest, Harvard University, Petersham, Massachusetts.
Franklin, J.F., Berg D.R., Thornburgh D.A., Tappeiner J.C. (1997) Alternative silvicultural approaches to timber harvesting: variable retention harvest systems. Creating a Forest for the 21st Century: the Science of Ecosystem Management. Island Press, Washington, DC: 111-139.
Franklin, J.F., Spies, T.A., Van Pelt, R., Carey, A.B., Thornburgh, D.A., Berg, D.R., Lindenmayer, D.B., Harmon, M.E., Keeton, W.S., Shaw, D.C. (2002) Disturbances and structural development of natural forest ecosystems with silvicultural implications, using Douglas-fir forests as an example. Forest Ecology and Management, 155(1-3), 399-423.
Franklin, J.F., Mitchell R.J., and Palik B.J. (2007) Natural disturbance and stand development principles for ecological forestry. Gen. Tech. Rep. NRS-19. Newtown Square, PA: US Department of Agriculture, Forest Service, Northern Research Station. 44 p.
Grier, C.C., and Logan R.S. (1977) Old‐growth Pseudotsuga menziesii communities of a western Oregon watershed: biomass distribution and production budgets. Ecological Monographs 47.4: 373-400.
Gu H. and Bergman R. (2018) Life Cycle Assessment and Environmental Building Declaration for the Design Building at the University of Massachusetts. U.S. Forest Service Forest Products Laboratory FPS-GTR-255.
Hadley J.L. and Schedlbauer J.L. (2002) Carbon exchange of an old-growth eastern hemlock (Tsuga canadensis) forest in central New England. Tree Physiology 22, 1079–1092.
Hamburg, S.P., Vadeboncoeur M.A., Johnson C.E., and Sanderman J. (2019) Losses of mineral soil carbon largely offset biomass accumulation 15 years after whole-tree harvest in a northern hardwood forest. Biogeochemistry (2019) 144:1–14. https://doi.org/10.1007/s10533-019-00568-3.
Hanson, J.J., Lorimer, C.G., Halpin, C.R., Palik, B.J. (2012) Ecological forestry in an uneven-aged, late-successional forest: Simulated effects of contrasting treatments on structure and yield. Forest Ecology and Management 270: 94-107.
Page 9 of 11
Harmon, M.E., Ferrell W.K., and Franklin J.F. (1990) Effects on carbon storage of conversion of old-growth forests to young forests. Science 247.4943: 699-702.
Harmon, M.E., and Marks B. (2002) Effects of silvicultural practices on carbon stores in Douglas-fir western hemlock forests in the Pacific Northwest, USA: Results from a simulation model." Canadian Journal of Forest Research 32.5: 863-877.
Harmon, M.E., Moreno, A., Domingo, J.B. (2009) Effects of partial harvest on the carbon stores in Douglas-fir/western hemlock forests: a simulation study. Hoover C. and Stout S. (2007) The Carbon Consequences of Thinning Techniques: Stand Structure Makes a Difference. J Forestry 105(5):266–270. Hurteau, M.D., and Brooks, M.L. (2011) Short- and Long-Term Effects of Fire on Carbon in US Dry Temperate Forest Systems. BioScience 61 (2): 139–46. https://doi.org/10.1525/bio.2011.61.2.9. Iverson, Louis R., and Anantha M. Prasad (2001) Potential changes in tree species richness and forest community types following climate change. Ecosystems 4.3: 186-199. Janowiak, Maria K., et al. (2018) New England and northern New York forest ecosystem vulnerability assessment and synthesis: a report from the New England Climate Change Response Framework project. Gen. Tech. Rep. NRS-173. Newtown Square, PA: US Department of Agriculture, Forest Service, Northern Research Station. 234 p. 173:1-234. Keeton, W.S. (2006) Managing for late-successional/old-growth characteristics in northern hardwood-conifer forests. Forest Ecology and Management 235.
Keeton, W.S., Kraft, C.E., and Warren, D.R. (2007) Mature and old growth riparian forests: structure, dynamics, and effects on Adirondack stream habitats. Ecological Applications 17(3): 852-868.
Keeton, W.S., Whitman, A.A., McGee, G.G., and Goodale, C.L. (2011) Late-successional biomass development in northern hardwood-conifer forests of the northeastern United States. Forest Science.
Kittredge, D.B. (2009). The fire in the East. Journal of Forestry April/May pp. 162-163. Liang, Yu, et al. (2018) How disturbance, competition, and dispersal interact to prevent tree range boundaries from keeping pace with climate change. Global change biology 24.1: e335-e351.
Lorimer, C.G. and White, A.S. (2003) Scale and frequency of natural disturbances in the northeastern U.S., implications for early-successional forest habitats and regional age distributions. Forest Ecology and Management 185: 41-64.
McGarvey, J. C., Thompson, J. R., Epstein, H. E., and Shugart Jr, H. H. (2015) Carbon storage in old-growth forests of the Mid-Atlantic: toward better understanding the eastern forest carbon sink. Ecology, 96(2), 311-317.
Mladenoff, D.J., and Pastor, J. (1993) Sustainable forest ecosystems in the northern hardwood and conifer forest region: concepts and management. Pages 145–180 in G. H. Aplet, N. Johnson, J. T. Olson, and V. A. Sample, editors. Defining Sustainability.
Page 10 of 11
Northeast State Foresters Association (2015) The Economic Importance of the Forest-based Economies of the States of Connecticut, Massachusetts & Rhode Island, https://www.nefainfo.org/publications.html.
Nunery, J.S. and Keeton, W.S. (2010) Forest carbon storage in the northeastern United States: Net effects of harvesting frequency, post-harvest retention, and wood products. Forest Ecology and Management 259(8): 1363-1375.
Oliver C.D., Nassar N.T., Lippke B.R., and McCarter J.B. (2014) Carbon, Fossil Fuel, and Biodiversity Mitigation with Wood and Forests, Journal of Sustainable Forestry, 33:3, 248-275.
Olofsson, P., Holden C.E., Bullock E.L., and Woodcock C.E. (2016) Time series analysis of satellite data reveals continuous deforestation of New England since the 1980s. Environmental Research Letters 11(6):1–8. https://iopscience.iop.org/article/10.1088/1748-9326/11/6/064002/pdf
Oswalt S.N., Smith W.B., Miles P.D., and Pugh S.A. (2018) Forest Resources of the United States, 2017: A technical document supporting the Forest Service 2020 RPA Assessment. Gen. Tech. Rep. WO97. Washington, DC: U.S. Department of Agriculture, Forest Service, Washington Office. 237 pp.
Patterson, W.A., and Sassaman K.E. (1988) Indian Fires in the Prehistory of New England. In Holocene Human Ecology in Northeastern North America, edited by George P. Nicholas, 107–35. Interdisciplinary Contributions to Archaeology. Boston, MA: Springer US. https://doi.org/10.1007/978-1-4899-2376-9_6.
Proceedings of the National Academy of Sciences Apr 2009, 106 (15) 6082-6087
Puettmann, K.J., Coates K.D., and Messier C.C. (2009) A critique of silviculture: managing for complexity. Island Press.
Rhemtulla, J.M., Mladenoff D.J., and Clayton M.K. (2009). Historical forest baselines reveal potential for continued carbon sequestration.
Robertson, B., Lam F.C.F., and Cole R.J. (2012) A comparative cradle-to-gate life cycle assessment of mid-rise office building construction alternatives: Laminated timber or reinforced concrete. Buildings 2.3:245-270.
Rudell, S., Sampson R., Smith M., Giffen R., Cathcart J., Hagan J., Sosland D., Godbee J., Heisenbuttle J., Lovett S., Helms J., Priceand W. and Simpson R. (2007) The role for sustainably managed forests in climate change mitigation. J. Forestry 105: 314–319.
Russell, M.B., Woodall C.W., Fraver S., D’Amato A.W., Domke G.M., and Skog K.E. (2014). Residence times and decay rates of downed woody debris biomass/carbon in the Eastern United States. Ecosystems 17:765-777. Rustad, Lindsey, et al. (2012) Changing climate, changing forests: The impacts of climate change on forests of the northeastern United States and eastern Canada. Gen. Tech. Rep. NRS-99. Newtown Square, PA: US Department of Agriculture, Forest Service, Northern Research Station. 48 p. 99: 1-48.
Ryan, M.G., et al. (2010) A synthesis of the science on forests and carbon for US forests.
Page 11 of 11
Seymour, R.S., White, A.S., and Demaynadier, P.G. (2002). Natural disturbance regimes in northeastern North America—evaluating silvicultural systems using natural scales and frequencies. Forest Ecology and Management 155(1): 357-367. Stephenson, L., et al. (2014) Rate of tree carbon accumulation increases continuously with tree size. Nature 507.7490: 90-93.
Struck, D. (2019) Forget the Log Cabin. Wood Buildings are Climbing Skyward with Pluses Planet Washington Post Climate Solutions. https://www.washingtonpost.com/climate-solutions/2019/12/12/forget-log-cabin-wood-buildings-are-climbing-skyward-with-pluses-planet/
Swanson, F.J., and Chapin F.S. (2009) Forest systems: living with long-term change. Principles of Ecosystem Stewardship. Springer, New York, NY, pp 149-170. Tang, Guoping, and Brian Beckage (2010) "Projecting the distribution of forests in New England in response to climate change." Diversity and Distributions 16.1: 144-158.
Tollefson, J. (2017) The wooden skyscrapers that could help to cool the planet. Nature 545, 280–282.
Turner, M.G., Baker, W.L., Peterson, C.J, Peet, R.K. (1998) Factors influencing succession: lessons from large, infrequent natural disturbances. Ecosystems 1(6): 511-523.
Woodbury P. and Wightman J. (2017) Forest Management & Greenhouse Gas Mitigation Opportunities. Information Sheet #7, Cornell University.
Habitat for Birds
A white-eyed vireo perches high on a tree branch scanning the understory below. She’s searching for the perfect place to raise her young. The nesting site must match the shopping list inside her head: lush, impenetrable thickets of young trees and gnarly shrubs. She needs young trees at least three feet high, filled with nooks to build one small nest out of bark bits, leaves, and small roots all glued together with a sticky spider web. Green chunks of moss on the outside plug any unsightly holes. But this vireo will have a difficult time finding what she’s looking for in the Connecticut forest.
She is in search of a rare habitat in Connecticut, the young forest and shrublands. According to CLEAR’s Changing Landscape Project about fifty-nine percent of Connecticut is covered by forest, but only six percent of the forest is considered young or in an early stage of development. If we imagine that a habitat is like a room, then the Connecticut forest, which is mostly mature, is like a house with only one room. It would be like our white-eyed vireo spending all of her time lying on the couch. Wildlife like birds, small mammals, and salamanders depend upon having multiple rooms — a variety of habitats at different stages of growth — to fulfill their needs: one room to find food, one room to raise a family, and another to feel safe.
What Can Be Done?
Create a disturbance in the forest. What does that mean? Cut down some trees. It’s really that simple.* Historically, natural disturbances such as wind, fire, Native American agriculture, flooding, and beavers created enough young forest, fields, and shrublands to protect species diversity. But, today, we put out the fires, prevent the floods and restrict beaver activity. Humans must now actively create and manage young forest and shrubland habitat for those species that depend upon disturbance.
Woodland Owners: We Need You
Eighty-three percent of CT forest is owned by private individuals and families. If you are a woodland owner, you have an opportunity to create young forests and shrublands for wildlife. The problem is that cutting trees typically meets with a lot of resistance, mostly because it will result in some parts looking, well, like a mess. But this mess serves a purpose. The forest will pop back up in a year or two, creating that much-needed dining room or bedroom. Then the birds will arrive. Then more birds the next year, and still more the year after that.
Curious? If you would like to know more about creating a "mess" for wildlife on your property, please contact the UConn Extension Forestry
(thomas.worthley@uconn.edu) and tell us your story. We would be thrilled to talk to you about habitat projects.
To see what a young forest looks like in person, visit Sessions Woods Wildlife Management Area at 341 Milford St., Burlington, CT, where two young forest habitats were created in 2001 and 2013.
*Of course, always contact a certified forester to plan forest management activities
Image Source: Shutterstock.com. White-eyed vireo.